Phytoremediation with Salvinia minima (Salvineaceae): identification of temporal biomarkers during Cr(VI) removal
DOI:
https://doi.org/10.31055/1851.2372.v59.n3.44742Keywords:
Biomarkers, heavy metals, remediation, SalviniaAbstract
Background and aims: Heavy metals pollution, particularly by hexavalent chromium, is a topic of global concern due to its high toxicity and environmental persistence. Phytoremediation emerges as a nature-based economic technology for the recovery of environments contaminated by metals. In the case of water bodies, aquatic macrophytes such as Salvinia minima Baker are excellent candidates as remedial species. To optimize its use, it is important the identification of efficient and reliable biomarkers. The objective of this work was to carry out a temporal analysis of different biochemical parameters to identify both biomarkers of exposure to Cr(VI) and the most appropriate time for their analysis in S. minima plants under laboratory conditions.
M&M: Specimens of S. minima were cultured in the presence or absence of hexavalent chromium for seven days under controlled light and temperature conditions. Tissue and culture solution samples were taken at 0, 2, 4 and 7 days. The levels of H2O2, malondialdehyde, soluble and insoluble phenols and sucrose were quantified in floating pinnae and submerged rachis. Remaining Cr(VI) was determined in the treatment solution. All determinations were performed spectrophotometrically.
Results: The results allowed us to identify, among the selected parameters, early (malondialdehyde), medium-term (sucrose and insoluble phenols) and late (hydrogen peroxide) biomarkers.
Conclusions: The physiological implications of the observed variations are analyzed and discussed.
References
ADREES, M., S. ALI, M. IQBAL, S. A. BHARWANA, …& M. RIZWAN. 2015. Mannitol alleviates chromium toxicity in wheat plants in relation to growth, yield, stimulation of anti-oxidative enzymes, oxidative stress and Cr uptake in sand and soil media. Ecotoxicol. Environ. Saf. 122: 1-8. https://doi.org/10.1016/j.ecoenv.2015.07.003
AHMAD, P., D. K. TRIPATHI, R. DESHMUKH, V. P. SINGH & F. J. CORPAS. 2019. Revisiting the role of ROS and RNS in plants under changing environment. Environ. Exp. Bot. 161: 1-3https://doi.org/10.1016/j.envexpbot.2019.02.017
ANAND, S., S. K. BHARTI, N. DVIWEDI, S. C. BARMAN & N. KUMAR. 2017. Macrophytes for the reclamation of degraded waterbodies with potential for bioenergy production. En: KULDEEP, B., B. SINGH & J. KORSTAD (eds.), Phytoremediation Potential of Bioenergy Plants, pp. 333-351. Springer, Singapore.
ASSABGUI, R. A., L. M. REID, R. I. HAMILTON & T.ARNASON. 1993. Correlation of kernel (E)-ferulic acid content of maize with resistance to Fusarium graminearum. Phytopathology 83: 949-953.
AWA, S. H. & T. HADIBARATA. 2020. Removal of heavy metals in contaminated soil by phytoremediation mechanism: a review. Water Air Soil Pollut. 231: 47 http://doi.org/10.1007/s11274-016-2137-x
AZUBUIKE, C. C., C. B. CHIKERE & G. C.OKPOKWASILI. 2016. Bioremediation techniques classification based on site of application: principles, advantages, limitations and prospects. World J. Microbiol. Biotechnol.11: 180. https://doi.org/10.1007/s11274-016-2137-x
BHALERAO, S. & A. SHARMA. 2015. Chromium: As an
Environmental Pollutant. Int. J. Curr. Microbiol. App. Sci. 4: 732-746.
BRIFFA, J., E. SINAGRA & R. BLUNDELL. 2020.Heavy metal pollution in the environment and their toxicological effects on humans. Heliyon 6e0469https://doi.org/10.1016/j.heliyon.2020.e04691
CABANE, M., D. AFIF & S. HAWKINS. 2012. Lignins and abiotic stresses. Adv. Bot. Res. 61: 219-262. https:// doi.org/10.1016/B978-0-12-416023-1.00007-0
CARDINI, C., L. F. LELOIR & J. CHIRIBOGA. 1955. The biosynthesis of sucrose. J. Biol. Chem. 214: 149-155. https://doi.org/10.1016/S0021-9258(18)70953-8
ČESONIENĖ, L., E. MAŽUOLYTĖ-MIŠKINĖ, D. ŠILEIKIENĖ, K. LINGYTĖ & E. BARTKEVIČIUS.2019. Analysis of biogenic secondary pollution materials from sludge in surface Waters. Int. J. Environ. Res. Public Health 16: 4691. https://doi.org/10.3390/ijerph16234691
CHEN, S., R. LIN, H. LU, Q. WANG, … & C. YAN. 2020. Effects of phenolic acids on free radical scavenging and heavy metal bioavailability in Kandelia obovata under cadmium and zinc stress. Chemosfere 249: 126-341. https://doi.org/10.1016/j.chemosphere.2020.126341
CHO, U. & J. PARK. 2000. Mercury-induced oxidative stress in tomato seedlings. Plant Sci. 156:19.https://doi.org/10.1016/s0168-9452(00)00227-2
CHOCOBAR PONCE, S., C. PRADO, E. PAGANO, F. E. PRADO & M. ROSA. 2014. Effect of solution pH on the dynamic of biosorption of Cr(VI) by living plants of Salvinia minima. Ecol. Eng. 74: 33-41. https://doi.org/10.1016/j.ecoleng.2014.09.117
CHOCOBAR PONCE, S., C. PRADO, E. PAGANO, F. E.PRADO & M. ROSA. 2019. Effect of pH on Cr(III) accumulation, biomass production and phenolic profile in two Salvinia species. Environ. Toxicol. Chem. 38: 167-176. https://doi.org/10.1002/etc.4296
DEVI, S. S. & A. R. BISWAS. 2007. Heavy metal status and oxidative stress in diesel engine tuning workers of the central Indian population. J. Occup. Environ. Med. 49: 1228-1234.https://doi.org/10.1097/JOM.0b013e3181565d29 DHIR, B. 2013. Phytoremediation: Role of Aquatic Plants in Environmental Clean-Up. Springer, India. https://doi.org/10.1007/978-81-322-1307-9_2
DHIR, B., P. SHARMILA, P. P. SARADHI & A. A. NASIM. 2009. Physiological and antioxidant responses of Salvinia natans exposed to chromium- rich wastewater. Ecotoxicol. Environ. Safe. 72: 1790- 1797. https://doi.org/10.1016/j.ecoenv.2009.03.015
DIXIT, R., E. WASIULLAH, D. MALAVIYA, K. PANDIYAN, … & D. PAUL. 2015. Bioremediation of heavy metals from soil and aquatic environment: an overview of principles and criteria of fundamental process. Sustanability 7: 2189-2212. https://doi.org/10.3390/su7022189
DU, D. W. & J. BRAMLAGE. 1992. Modified thiobarbituric acid assay for measuring lipid oxidation in sugar-rich plant tissue extracts. J. Agr. Food Chem. 40: 1566- 1570.
ESPINOZA-QUIÑONES, F. R., A. N. MÓDENES, L. P. THOMÉ, S. M. PALÁCIO … & N. SZYMANSKI. 2009. Study of the bioaccumulation kinetic of lead by living aquatic macrophyte Salvinia auriculata. Chem. Eng. J. 150: 316-322.
https://doi.org/10.1016/j.cej.2009.01.004
ESPINOZA-QUIÑONES, F. R., E. A. DA SILVA, M. DE ALMEIDA RIZZUTTO, S. M. PALÁCIO, … & A. D. KROUMOV. 2008. Chromium ions phytoaccumulation by three floating aquatic macrophytes from a nutrient medium. World J. Microbiol. Biotechnol. 24: 3063- 3070. https://doi.org/10.1007/s11274-008-9853-9
FERRAT, L., C. PERGENT-MARTINI & M. ROMÉO. 2003. Assessment of the use of biomarkers in aquatic plants for the evaluation of environmental quality: application to seagrasses. Aquat. Toxicol. 2: 187-204. https://doi.org/10.1016/s0166-445x(03)00133-4
FLETCHER, J., N. WILLBY, D. M. OLIVER & R. S. QUILLIAM. 2020. Phytoremediation using aquatic plants. En: SHMAEFSKY, B. R. (ed.), Phytoremediation. In situ application, pp. 205-260. Springer, Cham.
FOYER, C. H. & G. NOCTOR. 2005. Oxidant and antioxidant signalling in plants: a re‐evaluation of the concept of oxidative stress in a physiological context. Plant Cell Environ. 28: 1056-1071. https://doi.org/10.1111/j.1365-3040.2005.01327.x
FRYZOVA, R., M. POHANKA, P. MARTINKOVA, H. CIHLAROVA, … & J. KYNICKY. 2018. Oxidative stress and heavy metals in plants. En: DE VOOGT, P. (ed.), Reviews of environmental contamination and toxicology, pp. 129-156. Springer, Cham. https://doi.org/10.1007/398_2017_7
FUENTES, I. I., F. ESPADAS GIL, M. C. TALAVERA, G. FUENTES & J. M. SANTAMARÍA. 2014. Capacity of the aquatic fern (Salvinia minima Baker) of accumulating high concentrations of nickel in its tissues, and its effects on plant physiological processes. Aquat. Toxicol. 155: 142-150. https://doi.org/10.1016/S0005-2736(00)00138-3
GARDNER, J. & S. AL-HAMDANI. 1997. Interactive effects of aluminum and humic substances on Salvinia. J. Aquat. Plant Manag. 35: 30-34.
GNIAZDOWSKA, A., U. KRASUSKA, K. CZAJKOWSKA & R. BOGATEK. 2010. Nitric oxide, hydrogen cyanide and ethylene are required in the control of germination and undisturbed development of young apple seedlings. J. Plant Growth Regul. 61: 75-84.
GONZALEZ, C. I., M. A. MAINE, J. CAZENAVE, G. C. SANCHEZ & M. P. BENAVIDES. 2015.
Physiological and biochemical responses of Eichhornia crassipes exposed to Cr (III). Environ. Sci. Pollut. Res. 22: 3739-3747. https://doi.org/10.1007/s11356-014-3558-4
GRATÃO, P. L., M. N. V. PRASAD, P. F. CARDOSO, P. J. LEA & R. A. AZEVEDO. 2005. Phytoremediation: green technology for the clean-up of toxic metals in the environment. Braz. J. Plant Physiol. 17: 53-64. https://doi.org/10.1590/S1677-04202005000100005
HAMA AZIZ, K. H., F. S. MUSTAFA, K. M. OMER, S. HAMA … & K. O. RAHMAN. 2023. Heavy metal pollution in the aquatic environment: efficient and low-cost removal approaches to eliminate their toxicity: a review. RSC Adv. 13: 17595-17610. https://doi.org/10.1039/D3RA00723E
HUANG, Z., X. D. PAN, P. G. WU, J. L. HAN & Q. CHEN. 2014. Heavy metals in vegetables and the health risk to the population in Zhejiang, China. Food Control 1: 248-252. https://doi.org/10.1016/j.foodcont.2013.08.036
KHAN, M. A., M. RAM, P. JHA, M. M. AHMAD, … & M. Z. ABDIN. 2011. Screening and detection of biomarkers in chickpea plants exposed to chromium and cadmium. J. Environ. Biol. 32:51-55.
KARTHIK, V., S. PERIYASAMY, I. J. BEULA & T. TEMESGEN. 2021. Restoration of contaminated agricultural soils. En: THAPAR KAPOOR, R., H. TREICHEL & M. P. SHAH (eds.), Biochar and its Application in Bioremediation, pp. 381-401. Springer, Singapore.
https://doi.org/10.1007/978-981-16-4059-9_18
KUMAR, V., J. SINGH & P. KUMAR. 2019. Heavy metals accumulation in crop plants: Sources, response mechanisms, stress tolerance and their effects. En: KUMAR, V., R. KUMAR, J. SINGH & P. KUMAR (eds.), Contaminants in Agriculture and Environment: Health Risks and Remediation, pp. 38- 57. Agro Environ Media, Haridwar. https://doi.org/10.26832/AESA-2019-CAE
LAM, P. K. S. & J. S. GRAY. 2003. The Use of Biomarkers in Environmental Monitoring Programme. Mar. Pollut. Bull. 46: 182-186. https://doi.org/10.1016/S0025-326X(02)00449-6
LAM, P. K. S. 2009. Use of biomarkers in environmental monitoring. Ocean Coast. Manag. 52: 348-354. https://10.1016/j.ocecoaman.2009.04.010
LAGADIC, L., T. CAQUET & F. RAMADE. 1994. The role of biomarkers in environmental assessment (5). Invertebrate populations and communities. Ecotoxicology 3: 193-208.
LEWIS, M. & G. THURSBY. 2018. Aquatic plants: test species sensitivity and minimum data requirement evaluations for chemical risk assessments and aquatic life criteria development for the USA. Environ. Pollut. 238: 270-280.
https://doi.org/10.1016/j.envpol.2018.03.003
LLATANCE OYARCE, W., J. EMILIANI, D. BERGARA, L. SALVATIERRA & L. PÉREZ. 2019. Caracterización de los mecanismos de fitorremediación de Salvinia sp. frente a la exposición a metales pesados y su impacto sobre la fisiología vegetal. Energeia 16: 37-45.
MANARA, A. 2012. Plant responses to heavy metal toxicity. En: FURINI, A. (ed.), Plants and heavy metals, pp. 27-53. Springer, Dordrecht. https://doi.org/10.1007/978-94-007-4441-7_2
MELACON, M. 1995. Bioindicators used in aquatic and terrestrial monitoring. En: HOFFMAN, D. J., A. R. BARNETT, G. A. BURTON Jr. & J. CAIRNS Jr. Handbook of Ecotoxicology, pp. 220-240. Lewis Publisher, Florida.
MENCH, M., J. P. SCHWITZGUEBEL, P. SCHROEDER, V. BERT & S. GUPTA. 2009.Assessment of successful experiments and limitations of phytotechnologies: contaminant uptake, detoxification and sequestration, and consequences for food safety. Environ. Sci. Pollut. Res. 16: 876-900. https://doi.org/10.1007/s11356-009-0252-z
MIRETZKY, P., A. SARALEGUI & A. F. CIRELLI. 2004. Aquatic macrophytes potential for the simultaneous removal of heavy metals (Buenos Aires, Argentina). Chemosphere 8: 997-1005. https://doi.org/10.1016/j.chemosphere.2004.07.024
MITHÖFER, A., B. SCHULZE & W. BOLAND. 2004. Biotic and heavy metal stress response in plants: evidence for common signals. FEBS Lett. 566: 1-5. https://doi.org/10.1016/j.febslet.2004.04.011
OLGUÍN, E. J., E. HERNÁNDEZ & I. RAMOS. 2002. The effect of both different light conditions and the pH value on the capacity of Salvinia minima Baker for removing cadmium, lead and chromium. Acta Biotechnol. 22: 121-131. https:// doi.org/10.1002/1521-3846(200205)22:1/2<121::AID- ABIO121>3.0.CO;2-F
OLGUÍN, E. J., G. SÁNCHEZ-GALVÁN & T. PÉREZ- PÉREZ. 2007. Assessment of the phytoremediation potential of Salvinia minima Baker compared to Spirodela polyrrhiza in high-strength organic wastewater. Water Air Soil Pollut. 181: 135-147.
PARIDA, P., K. B. SATAPATHY & A. MOHAPATRA. 2020. Phytoremediation potential of aquatic macrophyte Azolla pinnata R. Br. and Salvinia molesta Mitchell to remove chromium from waste water. Plant Arch. 20: 2595-2601.
PRADO, C., E. PAGANO, F. E. PRADO & M. ROSA. 2012. Detoxification of Cr(VI) in Salvinia minima is related to seasonal-induced changes of thiols, phenolics and antioxidative enzymes. J. Haz. Mat. 239-240: 355-361.
https://doi.org/10.1016/j.jhazmat.2012.09.010 PRADO, C., E. PAGANO, F. E. PRADO & M. ROSA.2013. Metabolic interconnectivity among alternative respiration, residual respiration, carbohydrates and phenolics in leaves of Salvinia minima exposed to Cr(VI). Environ. Exp. Bot. 87: 32-38. https://doi.org/10.1016/j.envexpbot.2012.10.005
PRADO, C., F. E. PRADO, E. PAGANO & M. ROSA. 2015. Differential effects of Cr(VI) on the ultrastructure of chloroplast and plasma membrane of Salvinia minima growing in summer and winter. Relationships with lipid peroxidation, electrolyte leakage, photosynthetic pigments, and carbohydrates. Water Air Soil Pollut. 226: 1-16. https://doi.org/10.1007/s11270-014-2284-3 PRADO, C., M. ROSA, E. PAGANO, M. HILAL & F. E. PRADO. 2010. Seasonal variability of physiological and biochemical aspects of chromium accumulation in outdoor-grown Salvinia minima. Chemosphere 81: 584-593. https://doi.org/10.1016/j.chemosphere.2010.08.033
PSOTOVÁA, J., J. LASOVSKÝB & J. VIČARA. 2003. Metal-chelating properties, electrochemical behavior, scavenging and cytoprotective activities of six natural phenolics. Biomed. Papers 147: 147-153. https://doi.org/10.5507/bp.2003.020
RAHMAN, M. M., K. S. ISLAM & Y. CHONGLING. 2010. Effect of chromium stress on antioxidative enzymes and malondialdehyde content activities in leaves and roots of mangrove seedlings Kandelia candel (L.) Druce. J. For. Environ. Sci. 26: 171-179.
REZANIA, S., S. M. TAIB, M. F. M. DIN, F. A.DAHALAN & H. KAMYAB. 2016. Comprehensive review on phytotechnology: Heavy metals removal by diverse aquatic plants species from wastewater. J. Hazard. Mat. 318: 587-599. https://doi.org/10.1016/j.jhazmat.2016.07.053
RIAZ, M. W., M. I. YOUSAF, Q. HUSSAIN, M. YASIR, & L. SHAH. 2023. Role of lignin in wheat plant for the enhancement of resistance against lodging and biotic and abiotic stresses. Stresses 3: 434-453. https://doi.org/10.3390/stresses3020032
ROSA, M., C. PRADO, G. PODAZZA, R. INTERDONATO, … & F. PRADO. 2009. Soluble sugars metabolism, sensing and abiotic stress. Plant
Signal. Behav. 5: 388-393.
ROSA, M., C. PRADO, S. CHOCOBAR-PONCE,E. PAGANO & F. E. PRADO. 2017. Effect ofseasonality and Cr(VI) on starch-sucrose partitioning and related enzymes in floating leaves of Salvinia minima. Plant Physiol. Biochem. 118: 1-10. https://doi.org/10.1016/j.plaphy.2017.05.014
RÜDEL, H. & K. TERYTZE. 1999. Determination of extractable chromium (VI) in soils using a photometric method. Chemosphere 39: 697-708.
SÁNCHEZ-GALVÁN, G., O. MONROY, J. GÓMEZ & E. J. OLGUÍN. 2008. Assessment of the hyperaccumulating lead capacity of Salvinia minima using bioadsorption and intracellular accumulation factors. Water Air Soil Pollut. 194: 77-90. https://doi.org/10.1007/s11270-008-9700-5
SHANKER, A. K., C. CERVANTES, H. LOZA-TAVERA & S. AVUDAINAYAGAM. 2005. Chromium toxicity in plants. Environ. Int. 31: 739-53. https://doi.org/10.1016/j.envint.2005.02.003
SHARMA, A., D. KAPOOR, J. WANG, B. SHAHZAD, … & D. YAN. 2020. Chromium bioaccumulation and its impacts on plants: an overview. Plants 9: 100. https://doi.org/10.3390/plants9010100
SHARMA, S. S. & K. J. DIETZ. 2008. The relationship between metal toxicity and cellular redox imbalance. Plant Sci. 14: 43-50. https://doi.org/10.1016/j.tplants.2008.10.007
SOLOMOS, T. & G. G. LATIES. 1975. The mechanism of ethylene and cyanide action in triggering the rise in respiration in potato tubers. Plant Physiol. 55: 73-78.
SWAIN, T. & W. E. HILLIS. 1959. The phenolic constituents of Prunus domestica. The analysis oftissues of the Victoria plum tree. J. Sci. Food Agr. 10: 63-68.
TIMALSINA, H., T. GYAWALI, S. GHIMIRE & S. R. PAUDEL. 2022. Potential application of enhanced phytoremediation for heavy metals treatment in Nepal. Chemosphere 306: 135581. https://doi.org/10.1016/j.chemosphere.2022.135581
URQUIAGA, I. & F. LEIGHTON. 2000. Plant polyphenol antioxidants and oxidative stress. Biol. Res. 33: 55-64.http://dx.doi.org/10.4067/S0716-97602000000200004 VAIOPOULOU, E. & P. GIKAS. 2020. Regulations for
chromium emissions to the aquatic environment in Europe and elsewhere. Chemosphere 254: 126876.
https://doi.org/10.1016/j.chemosphere.2020.126876 VELUSAMY, K., S. PERIYASAMY, P. S. KUMAR,G. RANGASAMY, … & D. V. NGUYEN VO.2022. Biosensor for heavy metals detection in wastewater: A review. Food Chem. Toxicol. 168: 113307. https://doi.org/10.1016/j.fct.2022.113307
WAKEEL, A., M. XU & Y. GAN. 2020. Chromium induced reactive oxygen species accumulation by altering the enzymatic antioxidant system and associated cytotoxic, genotoxic, ultrastructural, and photosynthetic changes in plants. Int. J. Mol. Sci. 21: 728. https://doi.org/10.3390/ijms21030728
WANG, Q., H. LI, Y. ZHANG, X. WANG, … & W.QU. 2019. Evaluations of submarine groundwater discharge and associated heavy metal fluxes in Bohai Bay, China. Sci. Total Environ. 695: 133873. https://doi.org/10.1016/j.scitotenv.2019.133873
WILSON, G. & S. AL-HAMDANI. 1997. Effect ofchromium (VI) and humic substances on selected physiological responses of Azolla caroliniana. Am. Fern J. 87: 17-27.
YADAV, S. K., A. A. JUWARKAR, G. P. KUMAR,P. R. THAWALE, … & T. CHAKRABARTI.2009. Bioaccumulation and phytotranslocation of arsenic, chromium, and zinc by Jatropha curcas L.: impact of dairy sludge and biofertilizer. Bioresour. Technol. 100: 4616-4622.
https://doi.org/10.1016/j.biortech.2009.04.062
YAMASAKI, H., Y. SAKIHAMA & N. IKEHARA.1997. Flavonoid-peroxidase reaction as a detoxification mechanism of plant cells against H2O2. Plant Physiol. 115: 1405-1412.
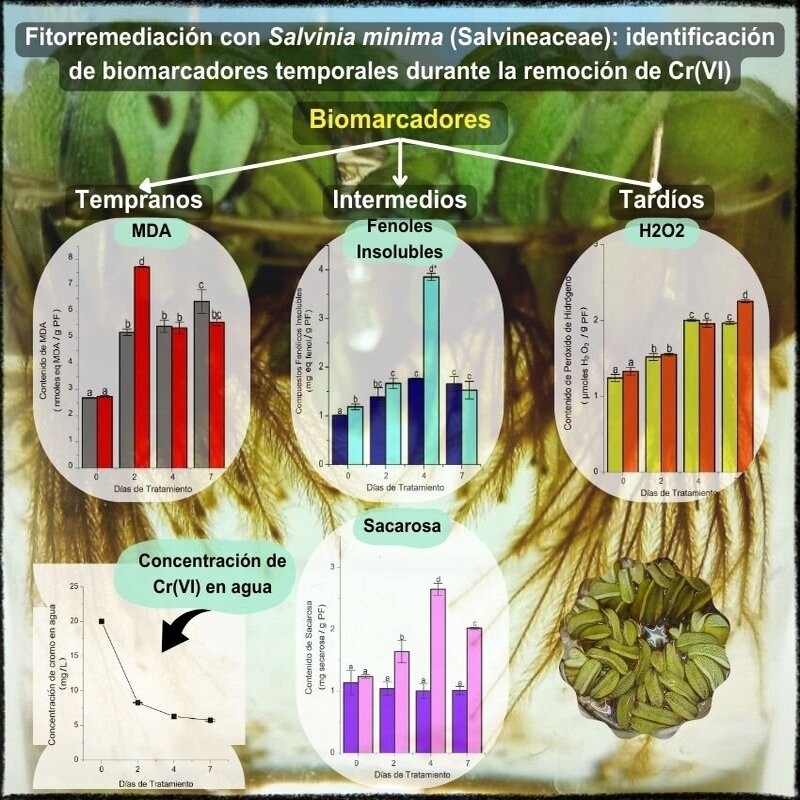
Downloads
Published
Issue
Section
License
Copyright (c) 2024 Noelia R. Tabernero, Silvana Chocobar Ponce, Carolina Prado, Mariana Rosa

This work is licensed under a Creative Commons Attribution-NonCommercial-NoDerivatives 4.0 International License.
Provides immediate and free OPEN ACCESS to its content under the principle of making research freely available to the public, which fosters a greater exchange of global knowledge, allowing authors to maintain their copyright without restrictions.
Material published in Bol. Soc. Argent. Bot. is distributed under a Creative Commons Attribution-NonCommercial-ShareAlike 4.0 International license.